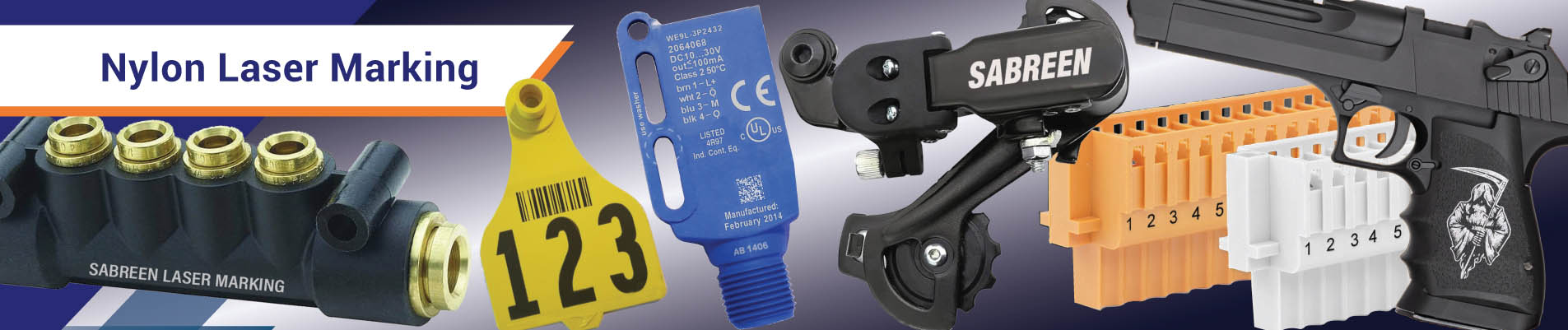
Nylon Polyamide Laser Marking, Engraving, Etching
How to Laser Mark Nylon Polyamide Plastics Using Fiber Lasers
Nylon plastics, also known as polyamide, are a prominent class of thermoplastics used in high-performance applications such as firearms, electronics, automotive manifolds, bicycle components, pipes, animal identification tags, sports shoe soles and more. They are among the most difficult polymers to laser mark, engrave, or etch due to their diverse material properties, molding conditions, and laser configurations. Ytterbium fiber lasers are ideal for producing black marking by carbonization, white marking by foam generation, and color changes by laser-induced thermal chemical reactions of pigmented polymers. Laser irradiance, fluence, and beam optics are important factors when marking plastics. Variable pulse MOPA fiber lasers offer the best pulse width control while limiting excessive heat.
Nylons are available in a variety of material types (e.g. Nylon 6,6; Nylon 6,12; Nylon 4,6; Nylon 6; Nylon 12 etc.), allowing for a diverse range of available materials and properties, whether homopolymer, co-polymer or reinforced. Long and short glass fibers are frequently incorporated in reinforced nylon. While advantageous for structural performance, the disadvantage is “glass emergence” defects from molding reduce laser markability.
Molded surfaces must be resin-rich so that energy from the laser can interact with the polymeric matrix. Nylons can also be blended with other engineering plastics to enhance specific performance characteristics. These factors inherently influence how each material type laser marks. Colors, pigments and dyes, filler properties, and compounding by various suppliers, molding dispersion and distribution, and surface texture are all factors that affect marking quality.
High-Quality Laser Marking Using Industrial Fiber Lasers: Functional and decorative indelible marking adds value to products while preventing counterfeiting. The key to achieving high-quality marking contrast and durability is to incorporate laser-enhancing additives into the nylon material during the molding process, whether with color concentrates or precompounded materials. Specialized additives designed for near-infrared lasers optimize marking contrast without affecting material properties, increase marking speed (shorter cycle time), and are cost-effective.
Industrial Laser Marking Experts
Single-Source Total Solutions for Plastics Laser Marking - Material Science through Custom Systems Integration.
Technical Services
- Custom laser color match formulations & compounds, regulatory compliance, prototype samples–masterbatch, pre-compounded materials (pre-color), laser laminates.
- Laser equipment systems design, turnkey systems integration & training, machine vision, custom HMI, process documentation.
- On-site problem-solving (any global location), laser process analysis & system optimization, custom vectorized artwork.
- Laser safety certification, complete application including design & CDRH Class 1 enclosure, laser safety officer (LSO) for internal audits.
- Plastics injection molding & analytical analysis, mold flow, additive-colorant dispersion/distribution.
- Job shop marking services
Laser Beam Quality: Ytterbium fiber lasers operate in the near-infrared (NIR) spectrum with wavelengths of 1060-1070 nm. The beam quality factor M2 is a quantification of the focus ability of a beam and is critical to marking performance. The M2 factor of a laser beam limits the degree to which the beam can be focused for a given beam divergence which is limited by the aperture of the focusing lens. M2 = 1 is the ideal Gaussian laser beam. Together with the optical power, the beam quality factor determines the brightness “radiance” of the laser beam. A consistent M2 factor is more important than the value itself, because different applications require different beam modes. Lower-performance lasers frequently suffer from a lack of consistency, which leads to marking variation, appearance, and functionality issues. Industrial lasers are superior to cheap wholesale (resellers) hobbyist-type lasers. Cheap lasers suffer from erratic marking quality, as well as inexpensive optics and electronics, resulting in variations in beam quality and inconsistent marking. All Lasers Are Not Created Equally.
Laser Marking, Engraving, and Machine Vision and Item Unique Identification (IUID): MIL-STD 130 is useful for inventory management and product tracking in a variety of industries. Poor marking quality is especially problematic and costly when 1D barcodes and 2D AIM DPM codes cannot be accurately verified and graded by the camera. Laser additives make a critical difference when high-resolution, line-edge quality, and small data cells are required for direct part marking (DPM) data matrix and bar code. The longer the data string, the more condensed the data cells become. Grade “A” verification (ISO 15415) is frequently required for military, aerospace, automotive and pharmaceutical products. High-contrast code readability provides additional benefits, such as faster marking speeds and the ability to use low-cost, hand-held readers throughout the factory.
Frequently Asked Questions About Laser Marking on Nylon
Why do some nylon materials produce better marking than others? While there are many theories about the pathway mechanisms of laser marking synthetic nylons there is no definitive answer. Some experts believe that nylons are distinguished by their amide groups (CONH). Amide groups foam more easily, which is due in part to the nitrogen, whereas methylene groups are better suited for carbonization. In PA12, the structure is more polyethylene-like, with an amide group every 12 monomer units. In contrast, PA6 contains an amide group every six monomer units. In practice, this means PA12 has a lower tendency to foam, and a higher tendency to produce dark marks than PA6. However, there are some exceptions to general observations.
Product design engineers must consider the performance of the part and its laser markability when selecting nylon.
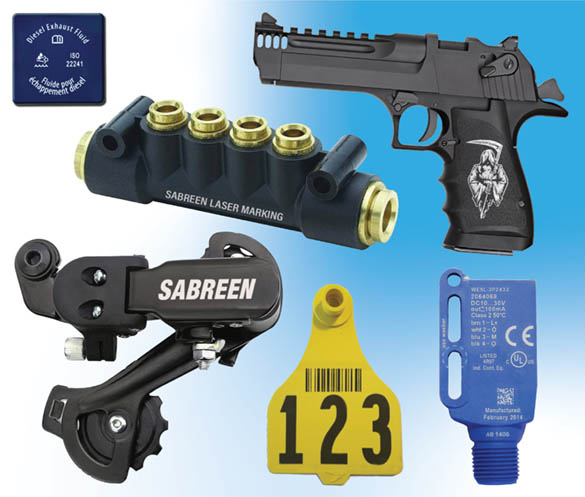
What are Nylon Polyamides?
Polyamides, also known as Nylon, are a prominent class of thermoplastics used in high-performance applications. They consist of amide linkages, specifically –CO-NH–. Nylon is synthesized through the process of polycondensation, wherein a diamine reacts with a diacid, or through ring-opening polymerization of lactams containing six, eleven, or twelve carbon atoms. Nylon monomers can be aliphatic, semi-aromatic or aromatic (aramids) and can range in structure from amorphous, semi-crystalline and of greater or lesser crystallinity. Two major polyamides are poly(hexamethylene adipamide) (nylon 6,6) and polycaprolactam (nylon 6). Aliphatic polyamides include nylon 12, 69, 6-10, 6-12, 46 and 1212. The bio-based engineering plastic known as Nylon 11 is sourced from renewable resources, specifically castor plants.
Do Fiberglass Additives Affect Laser Marking?
The quality of the marking depends on the polymer, additives and colorants, and the injection molding process. Molded polymer surface problems that inhibit readability can affect safety, performance, and function of the product. One example is additive glass fibers. The addition of glass fibers can deleteriously affect molding processes, which in turn affects the quality of laser marking. Fiberglass fill percentages vary, they typically range between 10 to 60%. There are two types of glass reinforcements – short fiber and long fiber and each has advantages and drawbacks. Molded part surfaces must be “resin-rich” in order to produce high-quality marking, whereby the energy from the laser interacts with the polymer matrix. Conversely, glass fiber-rich surfaces mark poorly, and the issue gets worse with higher fiber content. Fiberglass itself is not a good absorber of near-IR (NIR) laser light.
How Do Laser Enhancing Additives Work?
Since most polymers have limited absorption properties in this wavelength (NIR) and cannot produce noticeable contrast, experts use a variety of additives, fillers, pigments, and dyes to increase absorption and achieve high contrast quality. Additives increase the degree of color contrast (absorption of laser light), which is further enhanced by optimal laser selection and marking parameters. Unlike popular belief, there isn’t a single universal additive.
Some additives contain antimony-doped tin oxide and antimony trioxide mixtures, which can cause the natural (uncolored) substrate opacity to appear “grayish”. Other additives may contain aluminum particles, mixed metal oxides, or proprietary compounds. Color adjustments are made with pigments and dyes to achieve the desired colormatch appearance. Specific additives have been approved by the FDA for use in food contact and food packaging under conditions A-H of 21 CFR 178.3297 Colorants for Polymers.
Laser additive compounds are available in pellet granulate and powder form. Granulate products are blended directly with the polymer resin, while powder forms are converted into masterbatch. The loading concentration level by weight (in the final part) usually ranges between 0.01 and 3.0%. To achieve the best color contrast, laser additives must be distributed/dispersed uniformly throughout each part.
What are the Types of Polymeric Laser Marking Mechanisms?
Laser-markable plastics are characterized by their ability to absorb laser light and subsequently convert it into thermal energy within the near-infrared (NIR) range of wavelengths between 1060nm-1070nm. Three common types of lasers are Ytterbium fiber (MOPA & Fixed pulse), Vanadate (Nd:YVO4), and Nd:YAG. Depending on the type of laser and the desired marking contrast, a variety of chemistries can be used to produce surface reactions such as carbonization charring, chemical change foaming, and color change. The quality of the marking is dependent on the energy absorbed and the materials unique thermal degradation pathway. Each polymer grade, even within the same polymeric family, can produce different results. The same laser can perform all surface reactions by adjusting the settings in the marking software (power, pulse repetition rate, and marking speed). Continuous wave (CW) CO2 lasers have a wavelength of 10.6 microns (far infrared spectrum). CW CO2 lasers have lower peak power and cannot produce high contrast markings on plastics.
What is the difference between Fiber Lasers and Fiber-delivered Lasers?
Nanosecond Ytterbium fiber lasers are among the most significant advancements for marking, welding and cutting. Fundamentally, fiber lasers are different than other diode-pumped solid-state (DPSS) marking lasers. With fiber lasers, the active medium that generates the laser beam is dispersed within a specialized fiber-optic cable. In contrast to fiber-delivered lasers, the entire path of the beam is within a fiber-optic cable all the way to the beam delivery optics. This all-fiber structure is largely responsible for the reliability and ruggedness of these lasers, which accounts for their rapid growth.
How does Pulse Repetition Rate and Peak Power Affect Marking?
Pulse repetition rate and peak power density are critical parameters in forming the mark and achieving the optimal contrast and speed. High peak power at low frequency increases the surface temperature rapidly, vaporizing the material while conducting minimal heat into the substrate. As the pulse repetition increases, a lower peak power produces minimal vaporization but conducts more heat. The arithmetic curves of power versus pulse repetition rate are inversely proportional. Additional contributing factors of marking beam velocity and vector line separation distance determine the marking contrast and quality. By carefully adjusting and controlling these parameters, laser marking can be used to create precise, high-quality marks on a range of plastic materials. It’s a balance that depends on the material’s properties, the desired mark depth and the required marking speed. Adding laser absorbers to plastic greatly increases the range of markable plastic materials, improves the mark quality, and broadens the process window to insure mark consistency.
What is Laser Irradiance?
Laser irradiance: Irradiance is the power of the laser per unit area, measured in watts per square centimeter (W/cm2). It is essentially the intensity of the laser beam which is a function of the focused spot size. Focused laser spot size for any given focal length lens and laser wavelength is a function of laser beam divergence. Larger spot size results in lower energy density. High irradiance can lead to rapid heating and vaporization of the material, while low irradiance might not create a visible mark at all. The irradiance affects the speed and quality of the marking process. High irradiance can lead to faster marking, as the material is rapidly heated and vaporized. However, if the irradiance is too high, it can cause damage to the material, such as cracking or excessive burning. On the other hand, low irradiance may result in slow marking speed or no visible mark at all.
Intensity is influenced by:
- Pulse frequency (Hz, kHz) – pulse repetition rate
- Laser power (watt) – different from power density W/cm2
- Focal spot size – also referred to as focal diameter
- Pulse width – the time between the beginning and the end of an impulse measured in nanoseconds, pico-, or femtoseconds. A factor for controlling energy density, and critical for process optimization. Pulse duration adjustment in MOPA lasers is more flexible than Q-switched (fixed pulse) fiber lasers.
What is Laser Fluence?
Laser fluence: Fluence is a measure of the laser energy per unit area. It is typically measured in joules per square centimeter (J/cm2). Fluence determines the amount of energy that is transferred to the material surface. If the fluence is too low, the material may not be affected at all. If it’s too high, the material can be damaged excessively, beyond the desired marking effect. The fluence must be at an appropriate level for the specific material being marked. Some materials may require higher fluence to absorb sufficient energy for a visible mark, while others may require lower fluence to avoid damage. The depth of the mark also can be influenced by the fluence; generally, higher fluence leads to deeper marks.
Fluence is influenced by:
- Beam intensity
- Scanner velocity
- Pulse overlap
- Focus beam diameter
What are the Advantages of MOPA Fiber Laser versus Q-switch Fixed Pulse?
The emergence of MOPA (Master Oscillator Power Amplifier) designed nanosecond Ytterbium fiber lasers is one of the most significant advancements in laser plastic marking. An industry-changing laser design now provides pulsed energy differently than traditional mirror/Q-switch cavities. The design utilizes “waveforms” as a laser tool for quick processing and fine pulse control. Graph 1 shows the 40 different colored waveform pulses from the TRUMPF TruPulse Nano MOPA fiber lasers. Each color in the graph displays a distinct MOPA pulse waveform that can be used to balance the fluence and irradiance to optimize plastic marking results. As with many competing technologies, there often is confusion and unintended misrepresentation of important terminology and distinctive factors.
A MOPA fiber laser, Diagram 1 amplifies a modulated low-power telecom-quality laser diode to produce precise high-power laser pulses. By using an internal pulse generator to modulate the low-power diode, the laser can selectively set, maintain, or adjust the pulse width and resulting pulse energy over a broad pulse modulation rate.

This architecture effectively decouples the laser parameters versus Q-switched and provides greater process optimization and repeatability for better and more consistent marking results. Standard MOPA fiber laser designs can exhibit similar beam quality (M2) variation issues as Q-switched fiber lasers. TRUMPF, a leading fiber laser manufacturer, offers a patented dual-stage GTWave fiber amplifier design that maintains consistent M2 over its specified pulse repetition range. Unlike MOPA fiber lasers, an actively Q-switched fiber laser works by disrupting the gain in the laser cavity, thus allowing gain build-up in the amplifier and high-power laser pulses to be emitted when the disruption is removed. Key parameters in Q switched fiber lasers are tightly coupled.
The diode current can be increased or decreased to change the output power. Increasing the pulse repetition rate should increase the average power, however, this is not the case with Q-switched fiber lasers as the pulse power decreases. Chart 2 shows power and repetition rates as a function of time. The M2 (beam quality) on Q-switched fiber lasers can vary considerably with any change in laser power and pulse repetition rate. Varying the M2 between 1.1 and 1.5 will result in a 2x change in focused power density which can dramatically change marking quality on plastics.
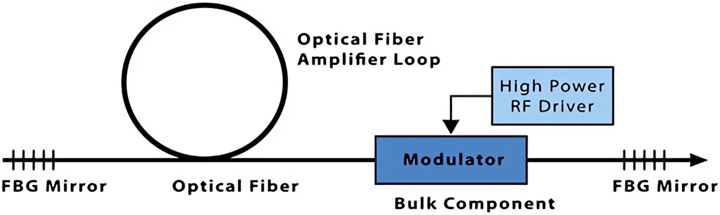
Applications for Laser Marking on Nylon
Nylon plastics have a wide range of applications including firearms, automotive, electrical & electronics, industrial, consumables and oil & gas. The most popular and challenging contrast colors are opaque white on black, jet black on white, and opaque white on medium blue.